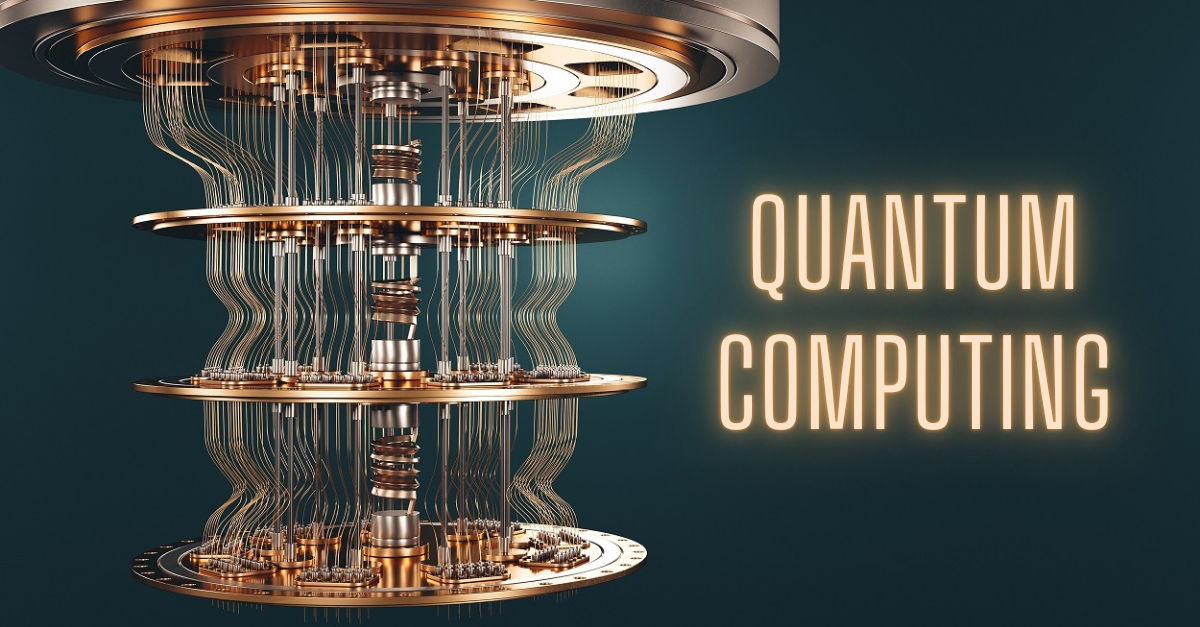
Table of Contents
Quantum computing, once a theoretical concept, is now on the brink of revolutionizing various sectors of our society. As this technology advances, it’s essential to understand its current state, potential applications, and the profound impact it may have on our daily lives.
Understanding The Future of Quantum Computing
Unlike classical computers that use bits to process information as 0s or 1s, quantum computers utilize quantum bits, or qubits. Qubits can exist in multiple states simultaneously, a phenomenon known as superposition. This allows The Future of Quantum Computing to perform complex calculations at unprecedented speeds, tackling problems previously deemed unsolvable by classical machines.
Current Developments in Quantum Computing
The Future of Quantum Computing In recent years grow significant, it made a quantum computing research and development. Leading technology companies and research institutions are investing heavily in building more stable and scalable quantum systems. For instance, IBM has been at the forefront, developing quantum computers and making them accessible for research and experimentation. Their efforts aim to push the boundaries of what’s possible in computation, opening new avenues for innovation.
Potential Applications of Quantum Computing
The unique capabilities of quantum computers position them to transform various industries:
- Healthcare and Drug Discovery
Quantum computing can simulate molecular structures and interactions with high precision, accelerating the development of new medications and treatments. This capability could lead to breakthroughs in understanding complex diseases and crafting personalized medicine approaches.
The healthcare sector is poised to benefit immensely from quantum computing. One of the most promising applications is in drug discovery. Traditional drug development is a lengthy and costly process, often taking years and billions of dollars to bring a new drug to market. Quantum computing offers the potential to accelerate this process by simulating molecular interactions more efficiently than classical computers.
For instance, quantum computers can model the behavior of molecules and predict how they will interact with various compounds, enabling researchers to identify promising drug candidates more quickly. This capability could revolutionize the way we develop medications, leading to faster and more cost-effective treatments for diseases.
Moreover, The Future of Quantum Computing can enhance precision medicine by analyzing complex genetic data to identify individual susceptibilities to diseases and tailor treatments accordingly. This personalized approach could improve patient outcomes and reduce adverse reactions to medications.
In recent years, there have been significant advancements in quantum computing technology. Companies like Google have announced plans to roll out commercial quantum computing applications within the next five years, focusing on areas such as materials science, drug development, and new energy solutions.
Additionally, collaborations between tech companies and healthcare institutions are paving the way for practical applications of quantum computing in medicine. For example, partnerships between quantum computing firms and pharmaceutical companies aim to utilize quantum algorithms to simulate molecular structures and interactions, potentially leading to the discovery of new drugs.
- Cryptography and Data Security
While quantum computers pose a threat to current encryption methods, they also pave the way for quantum cryptography. This new form of encryption leverages the principles of quantum mechanics to create secure communication channels, ensuring data integrity and privacy in the digital age.
Quantum computing is rapidly transitioning from theoretical research to practical applications, promising to revolutionize various sectors, including cryptography and data security. As this technology advances, it brings both unprecedented opportunities and significant challenges to the way we protect information. This article explores the current state of quantum computing, its implications for cryptography, and the proactive measures necessary to safeguard data in the quantum era.
At its core, quantum computing utilizes the principles of quantum mechanics to process information in ways that differ fundamentally from classical computing. Traditional computers use bits to represent data as 0s or 1s, whereas quantum computers employ quantum bits, or qubits, which can exist in multiple states simultaneously a phenomenon known as superposition. Additionally, qubits can be entangled, meaning the state of one qubit can be directly related to the state of another, regardless of the distance separating them. These properties enable quantum computers to perform complex calculations at speeds unattainable by classical computers.
Cryptography is the science of securing information, ensuring that data remains confidential, integral, and authentic. Modern cryptographic systems, such as RSA and ECC, rely on the computational difficulty of problems like factoring large prime numbers or solving discrete logarithms—tasks that are infeasible for classical computers to solve within a reasonable timeframe.
However, quantum computing poses a significant threat to these systems. Algorithms like Shor’s algorithm can efficiently solve these mathematical problems, rendering current encryption methods vulnerable. This means that data encrypted today could be decrypted by quantum computers in the future, compromising the confidentiality and integrity of sensitive information.
- Artificial Intelligence and Machine Learning
Integrating The Future of Quantum Computing with AI can enhance data processing capabilities, leading to more efficient algorithms and improved machine learning models. This synergy could result in smarter and more responsive AI applications across various sectors.
Quantum computing is revolutionizing the landscape of technology, and one of the most exciting areas of its application is in artificial intelligence (AI) and machine learning (ML). With the ability to process massive amounts of data at unparalleled speeds, quantum computing has the potential to redefine the way we approach AI-driven solutions. This article explores the latest developments in quantum computing, its implications for AI and ML, and what we can expect in the near future.
Traditional computers process information using binary bits (0s and 1s). Quantum computers, however, use quantum bits (qubits), which can exist in multiple states simultaneously due to the principles of superposition and entanglement. This allows them to perform complex calculations exponentially faster than classical computers.
Some of the key advantages of quantum computing include:
- Parallelism: The ability to process multiple possibilities at once, making computations significantly faster.
- Optimization: Quantum algorithms can solve complex optimization problems that would take classical computers years to process.
- Simulating Quantum Systems: Quantum computers can accurately model molecules and complex systems, leading to breakthroughs in chemistry, physics, and artificial intelligence.
AI and ML rely heavily on data processing, pattern recognition, and optimization. Quantum computing enhances these capabilities by:
1. Accelerating Machine Learning Algorithms
Traditional ML models require extensive computational resources, especially for tasks like deep learning and reinforcement learning. Quantum computing can accelerate these models by reducing the time needed for training and inference.
For instance, quantum-enhanced machine learning (QML) algorithms can process and classify large datasets exponentially faster, improving the performance of AI systems in areas like natural language processing, image recognition, and autonomous decision-making.
2. Enhancing Optimization in AI Models
Optimization is at the heart of machine learning, from hyperparameter tuning to neural network training. Quantum optimization algorithms, such as the Quantum Approximate Optimization Algorithm (QAOA), can significantly improve efficiency by quickly finding the best parameters for AI models.
3. Advancing Neural Networks and Deep Learning
Quantum computing enables the development of quantum neural networks (QNNs), which leverage quantum properties to improve learning efficiency. QNNs can potentially solve problems that classical deep learning struggles with, such as non-linearity and high-dimensional pattern recognition.
4. Revolutionizing Data Processing and Big Data Analysis
Quantum computing can handle vast amounts of unstructured data, extracting meaningful insights in record time. This capability is particularly useful in fields like finance, healthcare, and cybersecurity, where rapid data analysis is crucial.
- Logistics and Optimization
Quantum algorithms can solve complex optimization problems, such as route planning and supply chain management, more efficiently than classical methods. This can lead to cost savings, reduced environmental impact, and improved service delivery in industries like transportation and logistics.
Challenges Ahead
Despite its promise, quantum computing faces several hurdles:
Technical Challenges
Building stable qubits that can maintain coherence long enough for complex computations is a significant challenge. Additionally, developing error correction methods to ensure accurate results remains a critical area of research.
Ethical and Security Concerns
The power of quantum computers to break current encryption standards raises concerns about data security and privacy. It’s imperative to develop quantum-resistant encryption methods to safeguard sensitive information as this technology matures.
The Evolution of Quantum Computing: From Theory to Reality
Quantum computing has rapidly evolved from an abstract theoretical concept to a promising technology with the potential to revolutionize various industries, including cryptography, artificial intelligence, material science, and optimization problems. Unlike classical computing, which relies on bits (0s and 1s), quantum computing leverages the principles of quantum mechanics superposition, entanglement, and quantum interference to process information in fundamentally new ways. This article explores the journey of quantum computing, tracing its evolution from theoretical foundations to real-world applications.
Theoretical Foundations
The roots of quantum computing date back to the early 20th century with the birth of quantum mechanics. Pioneers like Niels Bohr, Werner Heisenberg, and Erwin Schrödinger laid the groundwork for understanding the quantum nature of particles. However, it was Richard Feynman and David Deutsch in the 1980s who introduced the idea that quantum mechanics could be harnessed for computation. Feynman famously proposed that simulating quantum systems would require a fundamentally different kind of computer a quantum computing.
Deutsch, in his seminal work, formalized the concept of a quantum Turing machine, demonstrating that quantum computation could solve certain problems exponentially faster than classical computers. This theoretical work set the stage for developing real quantum computing architectures.
Early Experiments and Algorithms
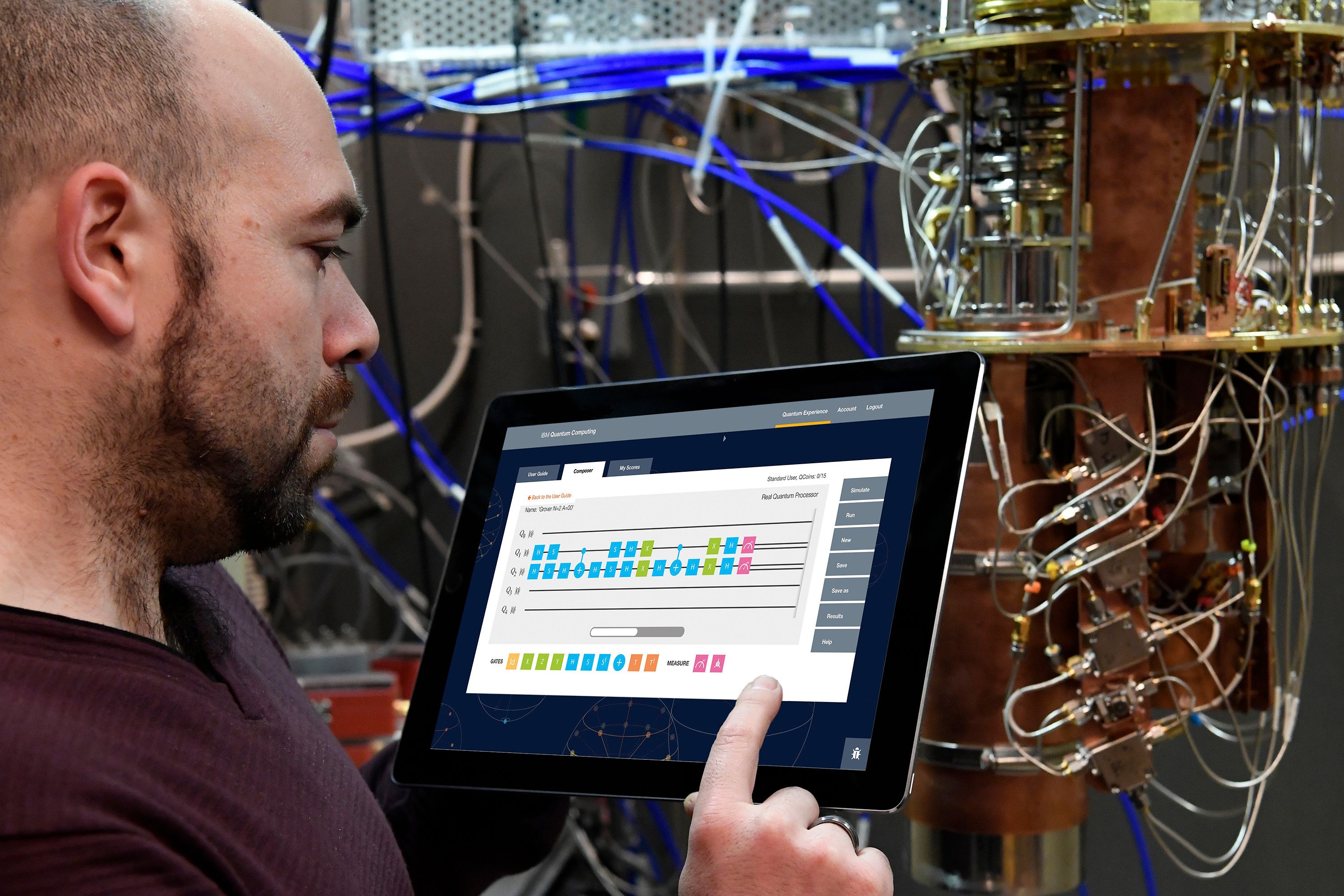
In the 1990s, researchers began translating quantum computing theory into practical experiments. The introduction of Shor’s algorithm in 1994 was a turning point it showed that a quantum computer could efficiently factor large numbers, posing a significant threat to classical cryptographic systems like RSA encryption. Around the same time, Grover’s algorithm demonstrated how quantum search algorithms could outperform classical search methods.
These breakthroughs spurred a surge of interest in quantum computing, leading to the first experimental implementations of quantum bits (qubits). The earliest qubits were built using nuclear magnetic resonance (NMR) in molecules, but these systems were limited in scalability.
Advancements in Quantum Hardware
The 2000s saw the emergence of more scalable quantum computing platforms. Researchers experimented with different qubit technologies, including:
- Superconducting qubits: Developed by IBM, Google, and Rigetti, these qubits use superconducting circuits cooled to near absolute zero to exhibit quantum behavior.
- Trapped ion qubits: Pioneered by researchers at the University of Maryland and IonQ, trapped ions are manipulated using lasers to perform quantum operations.
- Topological qubits: Microsoft has focused on this approach, which relies on exotic quasiparticles called anyons to provide more stable qubits.
- Photonic qubits: Companies like Xanadu and PsiQuantum are working on using photons to implement scalable quantum computing systems.
As quantum hardware improved, companies began building early quantum processors, with Google’s 2019 demonstration of quantum supremacy marking a major milestone. Google’s Sycamore processor performed a complex calculation in 200 seconds, which would take the best classical supercomputer thousands of years to complete.
Commercialization and Practical Applications
Today, quantum computing is transitioning from academic research to commercial viability. Major tech companies such as IBM, Google, Microsoft, and startups like D-Wave, IonQ, and Rigetti are racing to develop practical quantum systems. IBM introduced the first quantum computer accessible via the cloud in 2016, enabling researchers worldwide to experiment with quantum algorithms.
Some promising applications of quantum computing include:
- Cryptography: Quantum computers pose a risk to current encryption methods, but they also offer new cryptographic protocols, such as quantum key distribution (QKD), which ensures ultra-secure communications.
- Drug discovery and material science: Quantum simulations can model molecular interactions with unprecedented accuracy, accelerating the development of new materials and pharmaceuticals.
- Optimization problems: Quantum computing offers solutions for complex optimization challenges in logistics, finance, and supply chain management.
- Artificial intelligence: Quantum machine learning algorithms could significantly enhance pattern recognition and data processing capabilities.
Challenges and the Future
Despite significant progress, quantum computing still faces major challenges. The primary obstacles include:
- Qubit stability: Quantum states are extremely fragile, and maintaining coherence over time is difficult due to decoherence and noise.
- Error correction: Quantum error correction remains a major hurdle, as even slight errors can disrupt calculations.
- Scalability: Building large-scale quantum computers with millions of qubits is still an ongoing challenge.
However, researchers are making strides in overcoming these obstacles. The development of fault-tolerant quantum computers and hybrid quantum-classical systems suggests that practical quantum advantage may be achievable in the next decade.
Conclusion
Quantum computing has come a long way from its theoretical origins to tangible hardware and applications. While still in its early stages, the field is advancing rapidly, with major breakthroughs expected in the coming years. As technology matures, quantum computing promises to unlock unprecedented capabilities, transforming industries and reshaping the way we solve complex problems. The quantum revolution is just beginning, and its full potential is yet to be realized.